Introduction to 3D Cell Culture: The Future of Medicine
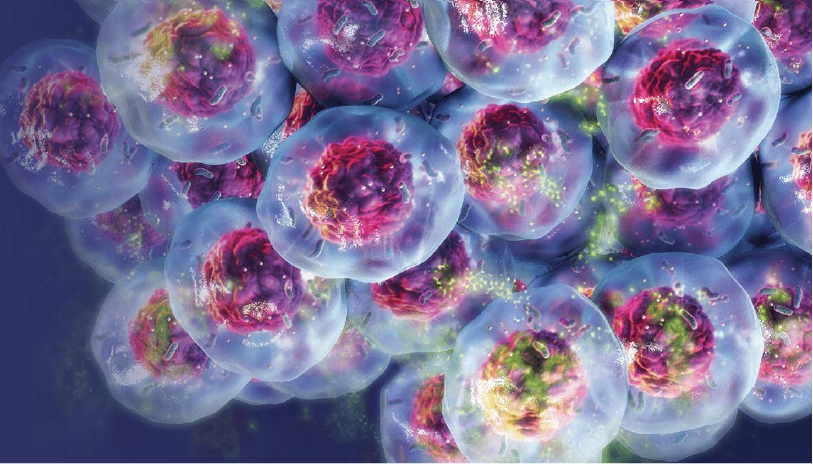
Three-dimensional (3D) cell culture is an advanced technique used in biological and medical research. Unlike traditional two-dimensional (2D) cell cultures that grow cells on a flat surface, 3D cell culture allows cells to grow and interact in all three dimensions. This provides a more realistic cellular environment that closely mimics natural tissues compared to standard flat culture methods.
History
The development of 3D Cell Culture techniques can be traced back to the 1980s. Some of the earliest methods involved growing cells on non-adherent surfaces or embedding them in reconstituted basement membrane proteins like Matrigel. Over the decades, a diverse range of 3D scaffolds, biomaterials, and microfluidic platforms have been developed to support 3D cell cultures. Advancements in tissue engineering, biomaterials, and biofabrication have greatly expanded the types of 3D culture models that can mimic complex tissue organization, morphology, and functions.
Benefits of Models
Compared to standard 2D cell monolayers, 3D cell cultures offer several physiological and technological advantages for biomedical research:
- Tissue-like architecture: 3D scaffolding allows cells to interact, organize, and self-assemble into multi-cellular structures that closely mimic natural tissues. This provides a much more realistic microenvironment for cell behavior studies.
- Improved cell viability: Cells generally survive longer and exhibit higher viability in 3D cultures compared to standard 2D monolayers. This is because 3D environments better support cell-cell communication, nutrient exchange, and waste removal.
- More accurate drug testing: 3D tissues are better models for predictive toxicology and pharmacology studies. They can assess drug response, differentiation, metabolism, and toxicity more accurately compared to simplified 2D systems. This leads to improved translation of preclinical research findings.
- Personalized models of disease: Patient-derived 3D organoids and organ-on-chip platforms allow modeling of human diseases and individual drug responses in a personalized manner close to the in vivo condition. This enables precision medicine approaches.
Applications
Recent innovations in 3D cell culture techniques have enabled the development of novel model systems that are being applied across diverse areas of biomedical research:
Cancer research: 3D tumor models recapitulate critical aspects of the tumor microenvironment and allow studying cancer development, progression, metastasis, and drug response more accurately compared to standard 2D methods. Organoid cultures derived from patient tumor biopsies are especially promising for personalized cancer therapies.
Tissue engineering: 3D cell culture is a key tool in developing tissue-engineered constructs for applications like drug screening, toxicology testing, and regenerative medicine. Scaffolds embedded with cells can be used to biofabricate tissues and organ models in vitro for transplantation.
Developmental biology: The spatial organization of embryonic tissues and organs can be better reconstructed using 3D culture techniques. This provides deeper insights into mechanisms of morphogenesis, stem cell differentiation, and developmental disorders.
Drug development: Advanced 3D cancer models, organoids, and organ-on-chip microfluidic devices show potential for accelerated preclinical drug development, toxicology assessment, and personalized medicine approaches with human tissues.
Microphysiological systems: Multi-organ microchips interfacing different tissue types via fluid flow allow modeling systemic physiology and complex organ-organ interactions better than individual tissue cultures. They can aid efficacy and safety testing of drug candidates.
Future Prospects and Challenges
While significant progress has been made, technology continues to evolve rapidly. Integration of inputs from fields such as stem cell biology, biomaterials engineering, microfabrication, and tissue engineering holds promise to advance the field in new directions. Some future opportunities and challenges include:
- Development of complex multi-tissue models interfacing multiple organ systems on chips to better recapitulate whole body physiology.
- Automation of processes like scaffold assembly, cell seeding and culture handling to enable high-throughput and industrial-scale applications.
- Integration of sensing, imaging and microfluidic capabilities in 3D culture platforms to enable real-time non-invasive monitoring of cellular dynamics.
- Derivation of advanced 3D tissue models using patient-specific induced pluripotent stem cells (iPSCs) for precision diagnostic and therapeutic applications.
- Overcoming limitations in nutrient/waste transport as model complexity increases with tissue size, which impacts cell viability in thick 3D constructs.
- Standardization and validation of novel 3D culture models and platforms for robustness and interlaboratory reproducibility.
3D cell culture is establishing itself as an indispensable tool for multiple areas of life sciences research and has promising implications for the future of medicine, biotechnology and human health. With continued advances, it holds strong potential to transform drug development, disease modeling, and regenerative therapies.
Get More Insights On, 3D Cell Culture
About Author:
Ravina Pandya, Content Writer, has a strong foothold in the market research industry. She specializes in writing well-researched articles from different industries, including food and beverages, information and technology, healthcare, chemical and materials, etc. (https://www.linkedin.com/in/ravina-pandya-1a3984191)
- Art
- Causes
- Crafts
- Dance
- Drinks
- Film
- Fitness
- Food
- Juegos
- Gardening
- Health
- Home
- Literature
- Music
- Networking
- Other
- Party
- Religion
- Shopping
- Sports
- Theater
- Wellness
